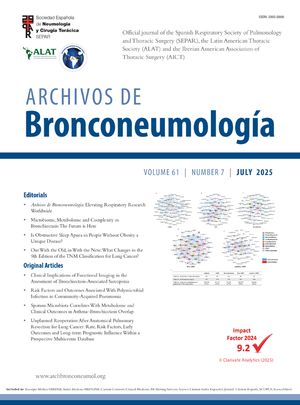
Lung cancer remains the leading cause of cancer-related deaths worldwide. According to the American Cancer Society (ACS), it ranks as the second most prevalent type of cancer globally. Recent findings have highlighted bidirectional gut–lung interactions, known as the gut–lung axis, in the pathophysiology of lung cancer. Probiotics are live microorganisms that boost host immunity when consumed adequately. The immunoregulatory mechanisms of probiotics are thought to operate through the generation of various metabolites that impact both the gut and distant organs (e.g., the lungs) through blood. Several randomized controlled trials have highlighted the pivotal role of probiotics in gut health especially for the prevention and treatment of malignancies, with a specific emphasis on lung cancer. Current research indicates that probiotic supplementation positively affects patients, leading to a suppression in cancer symptoms and a shortened disease course. While clinical trials validate the therapeutic benefits of probiotics, their precise mechanism of action remains unclear. This narrative review aims to provide a comprehensive overview of the present landscape of probiotics in the management of lung cancer.